Gravity
Article curated by Ed Trollope
The theory of gravity describes one of the most famous laws of physics. Mankind has known that things fall down since we were first able to think, and we've had a scientific explanation for the existence of gravity for several hundred years but, surprisingly, there are still more Things We Don't Know about gravity than physicists can shake an apple tree at.
Gravity is one of the few aspects of physics that is relevant at both the atomic and universal scales – and, at both ends, there's something wrong...
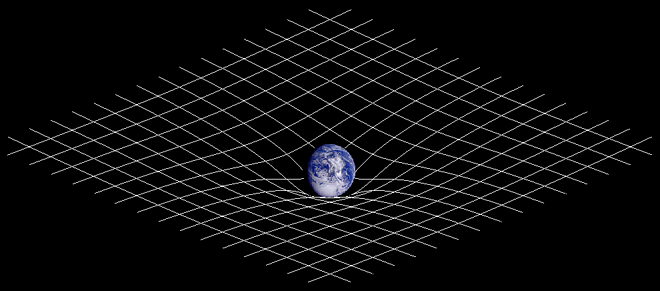
What's wrong with what we know?
At very small distances (much smaller than even the size of an atom), the laws of gravity should match up with those of relativity, but they don't. Unfortunately, we lack the technology to conduct measurements or observations on such a tiny scale, but several branches of theoretical physics are looking for the answer.

At much larger distances such as those between the planets of our solar system, our understanding of gravity is so good that we can launch spacecraft to land on other planets or visit asteroids and comets after journeys lasting many years. Yet there is evidence that our model isn't perfect on the interplanetary scale, and spacecraft don't always behave quite the way we expect them to. In fact, astronomers are finding more problems than they can shake a telescope at. These include: – The flyby anomaly, where spacecraft receive greater than predicted acceleration during gravity assisted manoeuvres. – Planetary orbits widening faster than expected if their sole cause is the sun losing mass by radiating energy. – Extra fast stars, which move faster than they should according to observed distributions of normal matter – Observed increasing rate of expansion of space. – Photons from the cosmic radiation background gain more energy than expected travelling through space, suggesting gravity falls off faster than usual at certain distance scales. – Extra massive hydrogen clouds – where hydrogen clumps together more than expected. – The weakness of gravity.


Even the speed of gravity is a matter of debate. We have several competing models of gravitation, and the speed of gravitational waves isn't the same in all of them. General relativity predicts that gravitational waves would travel at the same speed as light, and so far the experiments that have been done seem to support this – or at least, a speed very close to it. But those experiments are subject to controversy in themselves, so the question remains firmly unresolved.

The flyby anomaly
When planning missions into deep space, spacecraft missions planners sometimes don't want to rely entirely on thrust from engines. The spacecraft can flyby a body like a planet or moon, and use the pull of gravity to change course and accelerate it on its way. Unfortunately for the mission planners, the outcome of these manoeuvres isn't always what they expect – sometimes, but not always, the final velocity is a little bit different.
The flyby anomaly was first spotted in Dec 1990, when the NASA Galileo spacecraft performed such a manoeuvre around the Earth, on its way to Jupiter. In 1998, the anomaly struck again, this time with the NASA NEAR Shoemaker spacecraft[1], and was seen a third time in 2005 by the European
Learn more about The Flyby Anomaly.


Planetary orbits
Of course, when planning a mission to another planet, it helps if you know where the other planet is – and how far your spacecraft will have to travel. Because of the large distances involved in space, rather than talking about hundreds of thousands of kilometres, astronomers (and interplanetary spacecraft mission teams) use the Astronomical Unit (AU) instead. However, in 2004, Russian scientists claimed that the AU was increasing by roughly 1.5 cm each year, for unexplained reasons[2]. This may be related to planetary orbits widening faster than expected if their sole cause is the sun losing mass by radiating energy – something we can't explain.

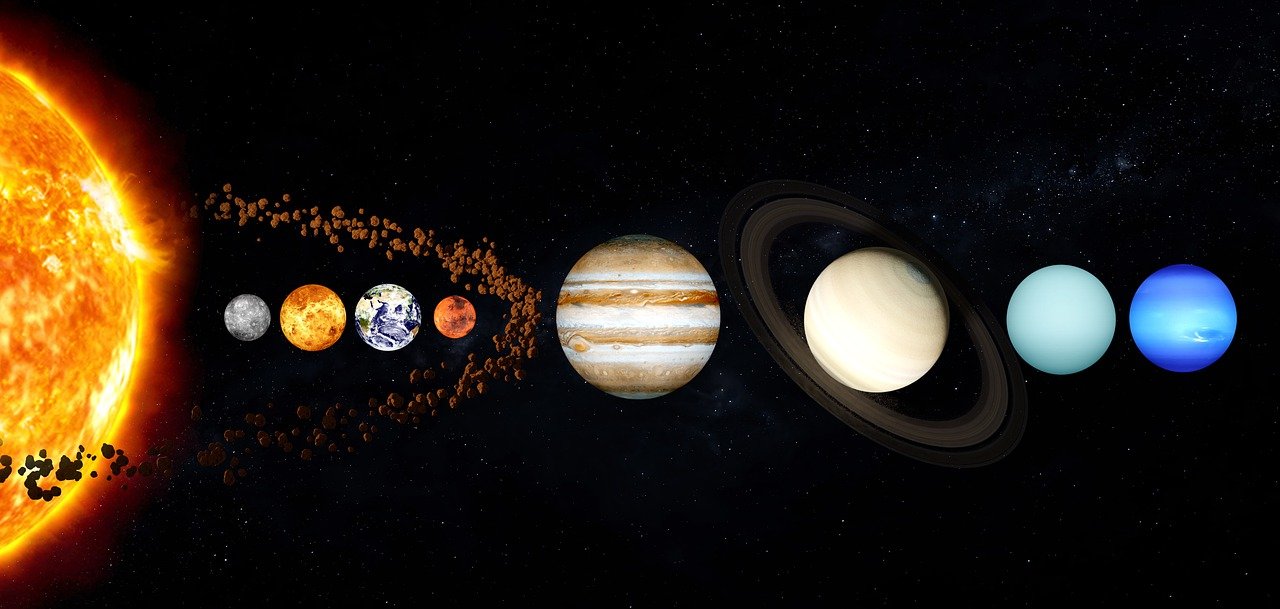
Let me play among the stars (extra fast stars)
If two people link arms and spin around in a circle as fast as they can, they don't fly apart. Stars are no different, and the stars in a galaxy are spinning around and around, held together by the force of gravity. The speeds of rotation of galaxies are related to their masses. We can infer the mass of a galaxy based on what we can 'see' of it. However, when observing galaxies in the universe, stars near the outside of galaxies appear to be moving faster than can be accounted for by the gravitational attraction of the mass of stars and dust we are able to see in them. In fact, they're moving so fast that gravity shouldn't be strong enough to stop them flying away.



The same is true of galaxies. They ought to fly apart from each other because their mass just isn't enough to keep them together. However, on an even larger scale, galaxies are organised into clusters: enormous collections of up to a thousand galaxies, bound by gravity. Again, the material we are able to detect directly indicates that the velocity of individual galaxies ought to decrease with distance from the cluster's centre. What we actually measure is that this value remains fairly constant. This indicates that our calculations of the galaxy's (or galaxy cluster's) mass based merely on what we can see are inaccurate, there must be something else that we cannot see.



Measurements so far have shown that the predicted motions of galaxies including the Milky Way, Andromeda, and other neighbouring galaxies deviate from the overall expansion of the universe by speeds of at least 600 km/s. Local effects such as the masses of nearby galaxies or voids may contribute to at least half of this motion! Scientists continue to investigate.


How do you weigh something, if you can only look at it from a long way away? This is a common problem for astronomers, who wish to know the masses of the stars and galaxies (and other more exotic objects) they're looking at, but astronomers have a number of ways of doing so (doppler effect, gravitational lensing, x-rays and the Sunyaev-Zel’dovich effect). But the problem is compounded when the object we want to measure is something we're inside – the Milky Way. In much the same way that we cannot see our own head without a mirror, we cannot look upon the Milky Way in the same way we would, say, Andromeda. So instead, astronomers look at objects that are orbiting the Milky Way, to determine what effect the galactic gravity is having upon them. Until recently, astronomers had estimated the weight to within a factor of about 4, but in 2015 a team of scientists used a new method to determine the mass to (they believe) within just 20%[3]. Knowing the mass of the Milky Way will be a big help in our efforts to understand how it formed and will evolve.




We call this dark matter.
Learn more about Dark Matter.

The gravitational lensing effect can take the appearance of an arc of light between a more distant object and closer, more massive object. Around some huge, distant, galaxy clusters, arcs like this have been seen that are orders of magnitude more prevalent than our best models of the evolution of the cosmos predict[5].Why this is remains a mystery.

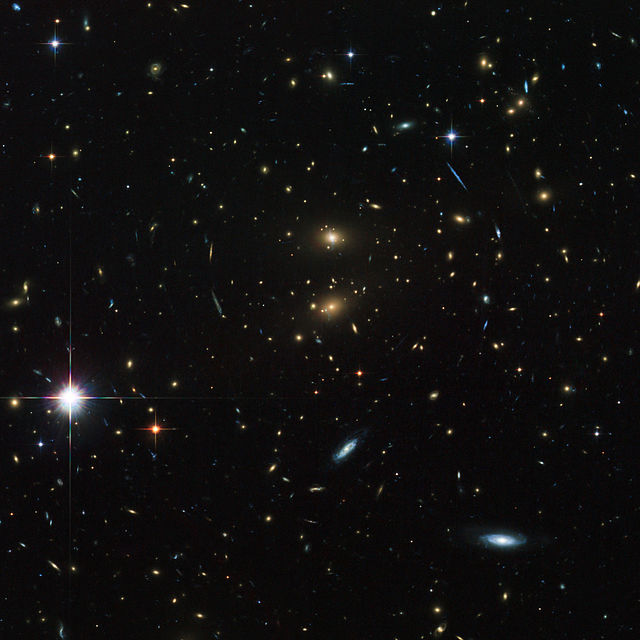
A dark matter
Dark matter has mass, but is not known to interact electromagnetically and so cannot be detected with telescopes, radio waves or x-rays. If this dark matter is indeed unaffected by electromagnetic forces, it may have been able to form structure in the universe before atoms had formed, and this hypothesis could explain the variations observed in the cosmic microwave background as well as the observed expansion of the universe as well as the observed relative abundance of elements created in the period shortly after the Big Bang.


The way in which stars are grouped in galaxies, galaxies in clusters and so on could be helpful to us in understanding dark matter and the mass of the particles responsible. This is because their mass would affect the speed at which they move compared to the speed of light, which in turn affects how structure would have formed in the early universe.


Dark matter is a substance we cannot see, but we can detect it by its gravitational effects. Antimatter, however, poses the opposite problem. Antiparticles are thought to possess mostly the same basic properties as ordinary matter (other than a few things like charge), but some properties, including the behaviour under gravity, have never been measured directly.
Researchers are able to produce large quantities of positronium – a bound state between an electron and positron (the electron's antimatter equivalent) which decays rapidly because the two components annihilate. The short lifetime of these atoms makes their properties particularly difficult to study, however, and they need to be able to extend the lifetime of positrons to determine the effect of gravity on them. Observing this could provide interesting clues to other questions regarding the expansion of the universe and the difference between quantities of matter and antimatter observed in the universe today.
Learn more about Properties of Antimatter.



The local void – a vast, unexplained empty region of space, squats just outside our galaxy and is virtually devoid of galaxies itself. Gravity dictates the matter is attracted to other matter, creating galactic clusters and filament structures – and so, by proxy, voids. And as matter is increasingly attracted to other matter, these matterless voids are expanding. Scientists now reckon that a point picked at random in the universe is more likely to be a void than a cluster. Our local void is probably the source of matter in our and neighbouring galaxies, and it could also teach us more about the existence and distribution of dark matter; however, there’s still a lot we don’t know about it, like why it’s where it is and how long ago it formed – but the local void has been notoriously tricky to study because it lies behind the central mass of our galaxy, the Milky Way blocking our view of this vast emptiness. However, an isolated dwarf galaxy found within it has allowed scientists some insight – including measuring the size (150 million light years diameter or more) and rate of expansion of the void (350 km/s).


Increasing expansion of space
Something must be pushing the universe apart, and we don't know what it is. Scientists do have some theories, but so far nothing matches what we can see.

Extra-energetic photons

The entropy of the universe is dominated by the cosmic microwave background, the radiative backdrop to the observable sky, an energy map of the early universe from shortly after the Big Bang when atoms formed and the universe stopped being opaque to light. Faint variations in this background radiation create a rippled energy map fossil of the early energy pathway – an anisotropic landscape of energy fluctuations, suggesting an early universe in thermal equilibrium, balanced at a maximum entropy before local variations led to clustering and the formation of planets and galaxies. But why? Surely it’s entropically unfavourable for the energy not to have spread out evenly? So, how did these variations form?
Scientists think that it’s all due to gravity. Gravity works differently to thermal energy because very spread out things have a very low rather than high gravitational entropy. When matter sticks together, it releases gravitational potential energy to its surroundings, allowing more disorder. So the gravitational entropy of the universe has increased, although thermal entropy has decreased. This must mean that gravitational entropy dominated the early universe, that or there are other entropic factors.
Learn more about /cosmic microwave background.



Analysis of the CMB data collected by the Planck Satellite and BICEP2 (a ground based telescope positioned in the South Pole) have revealed patterns in the polarisation of the radiation, giving the CMB the appearance of having a fingerprint, with swirls and arches. The polarisation of the radiation gives information about the medium through which the radiation has travelled, so this could be evidence for Einsteins hypothsised gravitational waves – remnants of the Big Bang, leading to clarification of Inflation Theory or even the nature of gravity itself. However, observations are tricky to make and the data iself has a low signal-to-noise ratio and so high percentage error.
Other astronomers have suggested that the polarisation patterns may be the result of dust in the interstellar medium scattering light and reflecting it, and may not be related to early universe theories at all. New and independant results have cast serious doubts on the original findings, supporting the space dust theory.
Learn more about /the big bang.


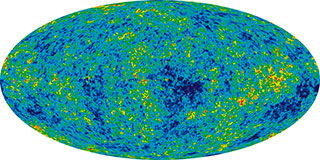
Extra massive hydrogen clouds
A series of absorption lines in the spectra of distant galaxies and quasars known as the Lyman-alpha forest suggest that hydrogen clouds are more clumped together at certain scales than expected and, like dark flow, may indicate that gravity falls off slower than inverse-squared at certain distance scales.


The weakness of gravity
The hierarchy problem describes the discrepancy between the strength of gravity and the strength of the weak force (which is 1032 times stronger). This problem can also be posed by asking why the Higgs boson is so light – surely they would stick to themselves and cause their mass to increase?
It is currently thought that after the Big Bang, the universe went through a period of inflation which caused it to be cold and flat. It has been suggested that, at this time, all of the energy of the Big Bang was “locked up” in a single type of particle: the reheaton. Then, as particles are wont to do, the reheatons decayed into lighter particles which make up the universe today.
Reheatons could decay into two particles: the Higgs we recognise and another particle (the remainder particle) to make up the rest of the energy. The reason that higher-mass Higgs bosons aren’t so prevalent is that, instead of the reheatons decaying to the higher-mass Higgs, they decay into smaller particles. As particles favour decaying into the fewest number of decay products, most of the energy from the Big Bang would end up as lighter Higgs rather than heavier Higgs, such that the types are unevenly represented. Although some physicists think this idea violates Occam’s razor, others think that some particles from higher-mass Higgs families were probably produced, and these could leave some evidence in the cosmic microwave background[6].
Learn more about /particles.


Black holes
Perhaps the most mysterious subject of the gravitational mysteries is black holes. Although their existence is now well documented, what lies at the centre of a black hole? Some have suggested a singularity – a point where a property is infinite. For black holes, this property is gravity. If you were to get pulled into a black hole, ignoring processes of spagettification (the process of being stretched out in a black hole due to the large gravity gradient – the force pulling on your feet is much stronger than that acting on your head), you'd reach the centre of infinitely strong gravity, and become infinitely dense. It is at this point that Einstein’s theory of general relativity breaks down and physics stops working. Another example of a singularity is the Big Bang with infinite density and infinite temperature. However, singularities produce numerous paradoxes where the laws of physics seem to fail.Research into loop quantum gravity and loop quantum cosmology – a combination of quantum mechanics and general relativity – has come up with a method that explains some of the issues with applying Einstein’s theory of general relativity to singularities by suggesting that what we think of to be singularities are actually gateways into another universe.
One research theory is that instead of this singularity, the universe emerged from a super dense mass with a history of its own, a Big Bounce rather than a Big Bang[7]. Another is that the information is somehow sent through to another universe. This theory would solve the information paradox surrounding black holes.
Learn more about Do Singularities exist?.


The information paradox states that if something goes into a black hole, the information is lost, which defies a fundamental law of physics stating information can only increase. Is there something wrong with this law? Or things pass through or escape black holes? Hawking radiation, energy or particles escaping a black hole and giving rise to asymmetry in matter and antimatter in our universe, has never been observed as any emission is swamped by dust or gas falling onto the black hole.

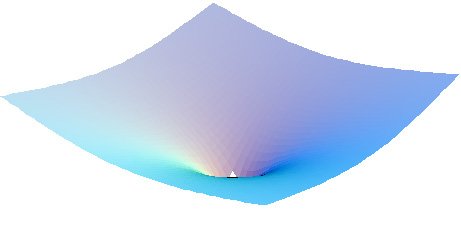
What is gravity, anyway?
According to the Standard Model, mass (and thus gravity) stems from the Higgs boson. Particles have mass because the Higgs boson 'sticks' to them. But this would imply that the bosons should also stick to each other, and get heavier and heavier – far heavier than the boson which was discovered at CERN, which is widely regarded to be the Higgs. So why don't Higgs particles stick together?
Learn more about Why don't Higgs particles stick together?.

All the fundamental forces of nature, apart from gravity, have been found to have associated elementary particles. Gravity is thought to also interact by a hypothetical elementary particle called the graviton. But, in order to prove the existence of gravitons, they would need to be observed using a particle detector – which is difficult to achieve because of the small scale at which gravitons are predicted to interact with matter, and the unfortunate fact that, if gravitons were detected, they would be indistinguishable from background neutrinos. For this reason there's not much current hope of answering the question "Are gravitons real?" There is, however, a lot of promise in measuring gravitational waves.

Learn more about Gravitational Waves.


The IceCube Neutrino Observatory, the largest neutrino telescope in the world, detected 28 neutrinos in 2013 that may have come from outside of the solar system[11].
Neutrinos only interact very weakly with matter, they pass straight through bodies such as the earth and they are also unaffected by gravity. Most neutrinos reaching the Earth originate from the sun or the Earth’s atmosphere, but some neutrinos (known as high-energy neutrinos) may originate from high energy cosmic events. As a result, these neutrinos could be the perfect tool for probing black holes and gamma-ray bursts.
Learn more about High-energy neutrinos.


Is gravity a fundamental force?
Learn more about Gravity and Thermodynamics.


Entropic gravity or emergent gravity describes this theory; in it, gravity is considered emergent and arises from the statistical behaviour of a large number of particles. The theory describes how macroscale homogeneity results as a consequence of microlevel disorder via the quantum entanglement of spacetime information. It is based upon string theory, black hole physics, and quantum information theory, and the system is framed through the lens of the second law of thermodynamics.
In the theory, because changing the arrangement of particles changes the amount of information needed to describe a system, it also changes the entropy. Matter affects the arrangement of particles, and so creates an entropic force – what we know as gravity.
The theory of entropic gravity is nearly impossible to test, however, because it predicts exactly the same gravitational behavior as general relativity. And where it differs, at the extremes of physics, it’s not really practical to test it, such as at gravitational field strengths below 1.2 × 10−10 m/s², one 80 billionth of the Earth’s. Some have tried to test Erik Verlinder’s theoretical equation for it, but this has its own problems: the equation was only intended to work in a narrow scenario, and many of the tests fall outside its scope.
Learn more about /entropy.



Other space objects
Where did the Martian moons come from? Mars is orbited by two small moons: Phobos and Deimos. These are small, relatively uneven bodies, and their origin is difficult to determine. It's possible that they are asteroids captured by Mars' gravity, or that they were ejected from Mars by some collision event, or even that they formed in orbit around the planet. But we don't know much about them, including many of their properties (notably their gravitational fields, and precise details of their composition), which could shed light on the origin of these objects.




Gravastars
A signal detected by one observatory, LIGO, could indicate the presence of a gravastar – a dense ball of matter kept inflated by a core of dark energy – something which has not been observed before[13]. The signal the observatory picked up was formed of three phases: the inspiral, the merger, and the ringdown. In the inspiral phase, the frequency of gravitational waves changes as though approaching a black hole; in the merger, the signal increases in both intensity and frequency; and in the ringdown phase, the signal rapidly drops off. It is thought that the structure of the ringdown can help astronomers differentiate between black holes and other objects because the ringdown may indicate the formation of an event horizon, as black holes are expected to be the only objects that will vibrate in this way. However, it might be possible for an object that is almost as compact as a black hole to vibrate in a similar way. One alternative is a gravastar. Instead of having an event horizon, gravastars can have a “light ring”, where photons are trapped in a circular orbit around the gravastar
.


From particle theorist to astronomers, physicists can’t escape gravity – and, with so many fundamental questions to answer, it could continue to exert its universal influence for some time.
This article was written by the Things We Don’t Know editorial team, with contributions from DLR, Ed Trollope, Jon Cheyne, Cait Percy, Johanna Blee, Grace Mason-Jarrett, Rowena Fletcher-Wood, and Alice Wayne.
This article was first published on 2015-08-27 and was last updated on 2021-07-19.
References
why don’t all references have links?
[1] P.G. Antreasian; J.R. Guinn (1998), Investigations into the unexpected delta-v increase during the Earth Gravity Assist of GALILEO and NEAR, AIAA/AAS Astrodynamics Specialist Conf. and Exhibition, Boston, paper no. 98-4287.
[2] Krasinsky, G. A.; Brumberg, V. A. (2004), Secular increase of astronomical unit from analysis of the major planet motions, and its interpretation, Celest. Mech. Dynam. Astron. 90 (3–4): 363, arXiv:1108.5546, Bibcode:2011CeMDA.111..363F, doi:10.1007/s10569-011-9377-8.
[3] Küpper, Andreas HW, et al. Globular cluster streams as galactic high-precision scales—The poster child palomar 5. The Astrophysical Journal 803.2 (2015): 80.
[4] Clowe, Douglas, et al. A direct empirical proof of the existence of dark matter. The Astrophysical Journal Letters 648.2 (2006): L109.
[5] Bartelmann, Matthias, et al. Arc statistics with realistic cluster potentials. IV. Clusters in different cosmologies. Astron. Astrophys. 330, 1–9 (1998).
[6] N. Arkani-Hamed, T. Cohen. R. T. D’Agnolo, A. Hook. H. D. Kim, D. Pinner. “Nnaturalness.” 2016. doi: 10.1103/PhysRevLett.117.251801.
[7] Ashtekar, A., Pawlowski, T., Singh, P., (2006) Quantum Nature of the Big Bang. Physical Review Letters 96(14). doi: 10.1103/PhysRevLett.96.141301.
[8] Abbott, B., et al., (2016) Observation of Gravitational Waves from a Binary Black Hole Merger. Physical Review Letters 116(6). doi: 10.1103/PhysRevLett.116.061102.
[9] Planck Collaboration Team (2014). Planck intermediate results. The angular power spectrum of polarized dust emission at intermediate and high Galactic latitudes. ArXiv. arXiv:1409.5738.
[10] Abbott, B., et al., (2016) GW151226: Observation of Gravitational Waves from a 22-Solar-Mass Binary Black Hole Coalescence. Physical Review Letters 116(24). doi: 10.1103/PhysRevLett.116.241103.
[11] IceCube Collaboration (2013). Evidence for High-Energy Extraterrestrial Neutrinos at the IceCube Detector. Science 342(6161):1242856. doi: 10.1126/science.1242856.
[12] Verlinde, E., (2011) On the origin of gravity and the laws of Newton. Journal of High Energy Physics 2011:29. doi: 10.1007/JHEP04(2011)029.
[13] Cardoso, V., Franzin, E., Pani, P., (2016) Is the Gravitational-Wave Ringdown a Probe of the Event Horizon? Physical Review Letters 116(17). doi: 10.1103/PhysRevLett.116.171101.
Blog posts about gravity





Recent gravity News
Get customised news updates on your homepage by subscribing to articles