Geoengineering
Article curated by Rowena Fletcher-Wood
We are pumping carbon dioxide into the atmosphere at an outrageous rate, and the climate is buckling under the pressure. Global temperatures are rising, leading to melting ice caps, storms and floods, loss of biodiversity and diminishing food supplies. So what can we do?
One answer is to stop doing it – stop putting the carbon dioxide out there in the first place – and the other is to stop the climate change directly – geoengineer the climate, rather than reduce emissions.
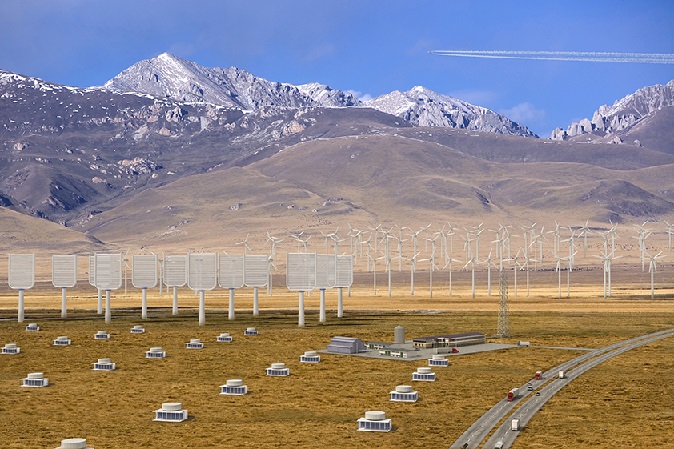
Geoengineering is a broad term that refers to doing anything big that mitigates worldwide anthropogenic climate change. Unhelpfully, this covers everything from reforestation to installing giant mirrors in space, but mostly when people talk about it, they mean the giant mirrors in space end of the spectrum, and other projects involving planetary scales and technology so new it’s still hugged in the crisp folds of bubble wrap.
The possibility of deliberately changing our climate is both rather exciting and damn scary. Any technology with global impacts is terrifying, even if they’re good impacts; the potential for exploitation, accident or unexpected consequences is completely unquantified. However, some argue that we’re already performing a dangerous global experiment with planet earth by emitting our greenhouse gases and, in August and September 2006, Paul Crutzen (Nobel Prize in Chemistry) and Tom Wigley (NCAR) recommended geoengineering as a viable emergency response.
We are living in the anthropocene – a time when humans can impact the environment around us so much that we leave a geological trace. Since the 1700s, when we switched from mostly burning wood to mining, we’ve become dependent on fossil fuels. We live in cities that are too big to go out and fetch wood from, and use up far more energy than we’d have time to collect. Worldwide, there are 50,000 fossil fuel power stations and more are being built every day – we’re far from freeing ourselves from unclean energy. And by the time our coal and oil reserves run out or become technologically inaccessible, it will be too late. That emergency I mentioned? It might be about to surface.



Carbon Capture and Storage (CCS)
The less we want our climate to change, the earlier we must stop burning fossil fuels. Carbon dioxide concentrations will continue to rise slowly for a while even after emissions stop because carbon dioxide is really long lived, hanging about in the atmosphere for hundreds of years, absorbing infrared radiation and converting it into molecular bending and stretching – that is, the vibrations we sense as heat. If we keep emitting carbon dioxide, the Earth will keep getting hotter. We need to average zero carbon dioxide emissions to stabilise the temperature at any value.
Stopping burning fossil fuels, farming cattle and giving out carbon dioxide entirely might seem impossible, but we can mitigate our actions using negative emissions technologies.
Some geoengineering is already happening: mitigation processes, such as planting trees, growing biofuels or breeding algae. But these are very different ideas from the new technologies the term brings to mind, so it is not always very helpful to talk about geoengineering, but to break down ideas into smaller categories, such as carbon capture and storage (CCS). Carbon capture and storage describes the removal of CO2 from the air, or collection of it at power stations. This carbon dioxide has to go somewhere, and instead of getting released could be locked away and stored underground or underwater. Estimates suggest up to 90% of our carbon dioxide outlet could be dealt with by CCS[1].
So what does CCS do? Carbon capture and storage includes tweaking ecosystems to enhance natural carbon dioxide removal processes – growing trees, for example, or fertilising the oceans – and technological fixes like pumping liquid carbon dioxide into the deep sea or porous rocks.
Ocean fertilisation. is the idea of bunging a lot of iron into the water to stimulate plankton growth and photosynthesis. In 2013, legal amendments made small scale ocean fertilisation experiments possible. However, results so far haven’t been great – plankton fix less carbon dioxide than expected, and their effectiveness varies massively between regions[2].
Iron is usually considered the limiting nutrient in phytoplankton growth, but nitrogen and phosphorous might also work. Theorists speculate that the photosynthesising plankton will eventually die, sinking to the ocean floor and storing the carbon there for 100 years. But is 100 years enough, and what will happen when it reemerges? Ocean fertilisation necessarily involves radically altering the ocean ecosystem. Marine biologists don’t know what this could mean, but at worse it could deplete oxygen in deep water, reduce biodiversity, introduce new toxic plankton species, and biochemically change fish. On the off-chance that the worst case scenario occurs, the UN Convention on Biological Diversity have ruled against large scale ocean fertilisation until we know more.
Carbon dioxide can be absorbed directly from the flue gas from power generation using spongy surfaces, or extracted from the air using massive CO2 scrubbers. It is definitely more efficient to collect the carbon dioxide at source than scrub it from the air later on: engineers calculate that it would take 10 million artificial trees to cut carbon dioxide levels by as little as 5 parts per million across a whole year. The principle of carbon collection at source was piloted from 2006 to 2014 at the Schwarze Pumpe, a high-CO2-emitting lignite-fired power station in Germany, where they successfully contained and liquefied carbon dioxide ready for storage. Unfortunately, the technology was considered too expensive to run longer.
Geological storage. So long as the natural oils, salt water or interstitial air can be pushed out and replaced, liquefied carbon dioxide may seep into rocks like sandstone to fill gaps between spherical grains. This process is known as geological sequestration. However, because stuff has to get pushed out, the injection procedure can involve high pressures that produce fracture risks – like fracking. Nevertheless, around 20 million tonnes of carbon dioxide is already stored safely in porous rocks in Norway, and the capacity for further geological sequestration is enormous: less than 1% of the available sandstone capacity would be filled by the output of 3 power stations over a 50 year period. On the other hand, we don’t know if carbon capture and storage would work long term without risk of leakage that could result in sudden, dangerous peaks in carbon dioxide levels. This could happen if earthquakes or slow geological changes over thousands of years damaged the integrity of storage rocks.



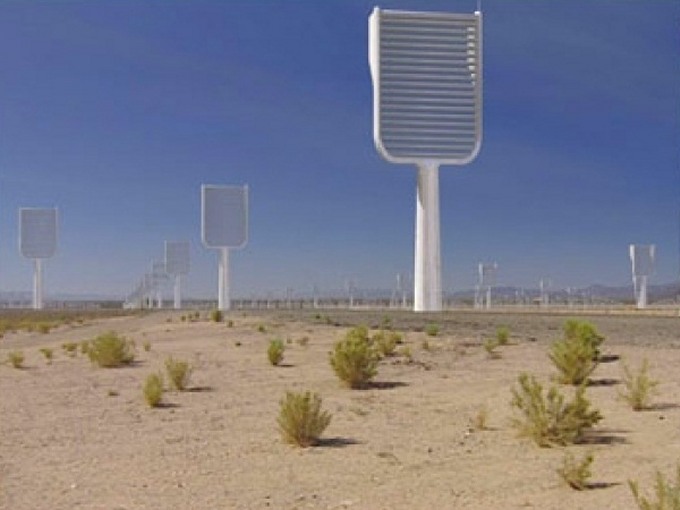
Ocean storage. The ocean is a mysterious place we don’t know a great deal about. It’s deep, and what happens down there where the pressure is very high is a bit enigmatic. It already removes as much as 40% of the carbon dioxide we eject into the atmosphere, which is why it’s becoming more acidic. But only if it dissolves near the surface. Below 3500 m, carbon dioxide turns to a liquid denser than water, and so becomes negatively buoyant, dropping rapidly to the ocean floor. This could provide a way to store carbon dioxide, but the method hasn’t been fully tested. The capacity for ocean storage is high: offshore in the UK alone, 78 billion tonnes of carbon dioxide could be stored at the bottom of the North Sea – 100 years worth of the stuff.
CO2 storage underwater could have an even larger impact on ecosystems and is filled with unknowns, not least because we still don’t understand the ecosystems at the bottom of the oceans and many of the animals and plants living there are yet to be discovered.


Bioenergy. Most of the Intergovernmental Panel on Climate Change’s (IPCC) < 2°C scenarios include negative emissions, and the best way of achieving these is considered to be BECCS (Bioenergy and CCS) – bioenergy and CCS. There are other similar ideas, such as fossil fuels and CCS (FECCS (Fossil Fuels and CCS)), but this involves zero emissions, whilst BECCS involve negative emissions. BECCS is a straightforward idea: grow crops that sequester carbon dioxide, then burn them in power stations, releasing the same carbon dioxide, which is captured and stored underwater or underground. This is oddly rather like going back to gathering and burning wood – but on an industrial scale[3].
In fact, it’s not just wood that would be grown and burnt, fast-growing, hardy, energy-rich plants like miscanthus are in the lineup for bioenergy. There’s only one problem: we can’t eat electricity. The areas of land required to grow all these plants for bioenergy would be huge (one estimate suggests increasing the US biofuel area by a factor of 20 could have the potential to remove a third of the emitted carbon dioxide a year[4]), and using land for fuel means using less land for crops, as well as using up massive volumes of water. This could have huge implications on world hunger, and domestic food prices would certainly rise.


Although research has increased in carbon capture and storage, most centres around governance, not whether it’s technically viable. CCS gets cheaper the more you do it, but to get it going there needs to be carbon tax that makes it more expensive to make carbon dioxide and do nothing than to mitigate it. Policy makers are conflicted over whether this should happen or not: carbon capture and storage doesn’t actually fix the “too much CO2” problem, just hides it away underground. This could make us complacent about cutting emissions, and increase the risks if CCS technologies ever failed or maxed out in the future. CCS is a bit like performing liposuction on an obese person: a quick fix for the right result that doesn’t address the actual problem.


Solar Radiation Management (SRM)
SRM involves using reflective surfaces to reduce the amount of solar radiation that gets absorbed. These surfaces could be placed out in space (like giant mirrors), squirted into the stratosphere (like aerosols of absorbent particles), established in the troposphere (cloud brightening) or on the surface of the planet (surface whitening). But reflection creates a cooling effect on the planet surface – it doesn’t do the opposite of adding carbon dioxide: carbon dioxide creates heating throughout the planet, not just at the surface. The downside of this is that if the reflectors are suddenly removed, global temperatures will rebound to what they would have been if solar radiation management had never been implemented. It also means that other consequences of high carbon dioxide levels, like ocean acidification and melting sea ice, will not be relieved.
Giant mirrors in space. At a place known as the L1-point, 1.5 km from earth, is a spot of equal gravity between the earth and the sun. It is here that scientists are deliberating placing a giant mirror 2000 km in diameter that could reflect up to 1% of the sun’s rays and create massive cooling. In theory, the mirror would just sit there, but in practice, it is likely to shift around a bit and require continuous adjusting. Another alternative is to use 16 trillion much smaller mirrors, which would be more challenging to coordinate, but significantly easier to engineer and launch.



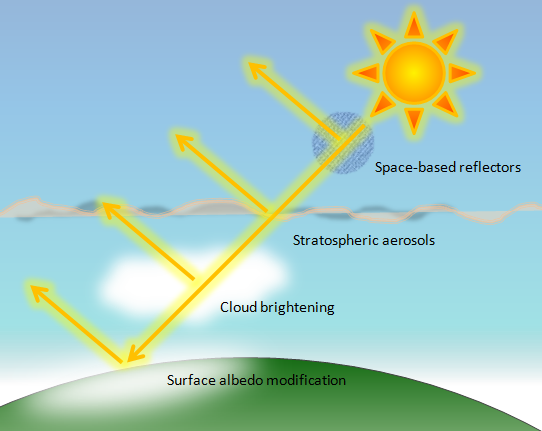
Stratospheric aerosols. Most of our understanding of solar radiation technology is based on volcanoes and what they do to our planet. The last eruption of Mount Pinatubo caused global cooling by emitting an aerosol of sulphate particles. For this reason, Stratospheric aerosols have received the most attention. However, natural processes like volcanic eruptions are still far from being safe! Of all the methods of solar radiation management, this one perhaps carries the greatest risk, but also the highest potential effectiveness.
Scientists have considered two ways of doing it: injecting particles into the tropical stratosphere and letting the wind do the job of distributing them, or just in the Arctic, where they could act to help reduce sea ice melt. The problem with this is that the particles are unlikely to stay above the Arctic indefinitely (there is wind there too), and the technology would need top ups as particles dissolved in rainwater, settled, or stuck together to form larger and less effective clumps.
In fact, the ideal particle would be as un-clumpy as possible, because small particles are good reflectors. The smaller the particles, the bigger the surface area compared to the volume; and it’s the surface that reflects and scatters light. Candidate particles include not only sulfur and its oxides, but also titanium dioxide (the stuff that makes paint white) and zinc oxide, calcium carbonate from limestone, silicates and aluminates like sand, or even diamond dust.



Cloud brightening, which really means cloud growing, is another way we could increase the solar reflectivity of the earth. Methods include cloud seeding, cirrus cloud thinning (these kinds of clouds trap more heat than they reflect light), or spraying more water particles into the atmosphere[5].
Albedo: the whiteness or reflectivity of the earth may also reduce solar radiation absorption. This could include whitening deserts, breeding plants with whiter leaves, fertilising the growth of reflective ocean algae, painting roofs and roads white, or floating billions of ping pong balls in the ocean. However, preliminary investigations suggest that these may be the least effective forms of geoengineering the climate, as well as a little bit peculiar[6][7].


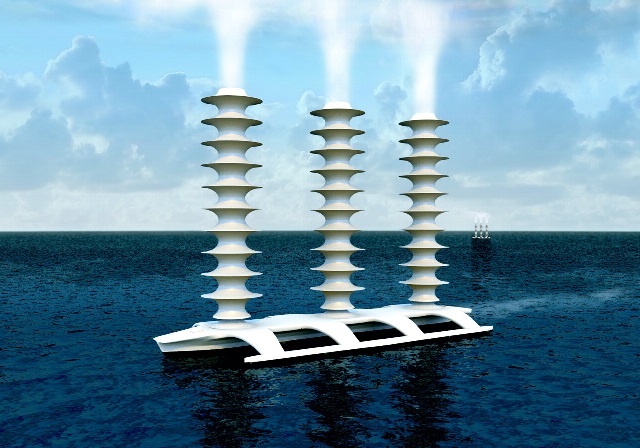
Sacrifices. Solar radiation management comes with some sacrifices. Like rainfall. Reducing solar radiation to keep temperature constant reduces precipitation, and you can’t control temperature and precipitation at the same time. They’re co-dependent. It will also provide uneven results: more cooling over the continents than the oceans, and differences between the continents that we can only make guesses at – in other words, winners and losers of the climate change game. Predictions suggest that the damage to the Amazon rainforests would be irreversible[8]. Some areas are going to continue getting warmer with climate change, like the north and south poles, and the deserts, which will get drier and spread, whilst other, more temperate areas will be less affected. This impacts food. In Africa and Asia, longer droughts will lead to more failed crops, smaller areas of arable land and, necessarily, hunger. The impact on ecosystems would be irreversible, especially in the oceans. We know that some animals, like whales, are depleted by increasing sea temperatures, and have a knock on effect decreasing other biodiversity – but so far we don’t know many species that do this, or how. Other environmental impacts include sunburn. Solar radiation management is likely to give us whiter skies with poorer stargazing and satellite communications potential, and greater exposure to the sun’s rays. But... beautiful sunsets[9]!
We might also expect damage to aeroplanes, and loss of solar electricity and heat. But worse than that, stratospheric aerosols will change the very chemistry of our atmosphere. Like volcanoes, shoving SO2 into the atmosphere produces a bit of NOx gases that affect its electrical behavior, and a lot of chlorine… and chlorine catalytically destroys ozone, leaving the planet less well protected against UV light. Calculations suggest that if 5 Tg of SO2 were to be injected every year, every year we’d lose ~1% of the minimum required ozone layer[10]. Changes in the kind and amounts of radiation the earth receives could also affect plant ecology, and possibly impact further up the food chain. In fact, we don’t know how SRM could affect biodiversity – we only know that climate change would affect biodiversity horrifically, irreversibly, and by wiping out a whole chunk of it – leading us to the conclusion that SRM is probably better – different, but better (and, like we said, with winners and losers).



A few guesses have been made about how SRM will affect our food. The way crops are affected by solar radiation management is generally opposite to the way they are affected by global warming. Some will thrive under the carbon dioxide enriched atmosphere, and some will wilt under the change in temperature. Tropical crops (and cotton) will generally be advantaged and temperate crops (like maize and soybeans) disadvantaged. Modelling rice suggested it pretty much would remain unaffected[11].


There are also socio-economic concerns around allowing that level of engineering to control our health, food, and wellbeing. "Whose hand is on the thermostat?" scientists ask. How can we protect our environment in the case of military or commercial conflict, societal disruptions, human errors, or changes in political ideology?
In an interesting phone call, Robock was asked whether, if some other country were trying to control the American climate, they would know about it. After a bit of thought, he said yes – clouds and other unusual atmospheric behavior would be very detectable. But after he put the phone down he had a rather different thought: that what they might really have been asking was if we wanted to control some other country’s climate, would they know about it?



So how can we improve our guesses and make better future predictions? One answer is climate modelling: using computers to minimise the human errors in our estimates and carry out the best risk assessments we can imagine. Recent workers in this area have focussed on making sure their models line up with each other. Afterall, it’s no use if NASA think the climate has another century and the Met Office are worried about making it through to Tuesday. If the models agree, we can be more confident, because a lot of different people are coming up with the same kinds of answers. Climate models are extremely sensitive to small details – like how much stuff to put out there, how big the particles are, and how fast they enter the troposphere.



Technology does not currently exist to implement solar radiation management tomorrow, nor is the technology cheap nor easy: but it’s possible. To squirt sulphate particles into the stratosphere, we would need massive guns, 800,000 aeroplanes for a decade, or huge exploding hydrogen balloons[12]. And the price of SO2 would be $50,000,000 a terogram, once ammunition, gun barrels, stations, and personnel was taken into account.


Although Carbon capture and storage seems like a modern invention, it isn’t. It’s been about since the dawn of time, when coal swamps absorbed large volumes of CO2, and rapid photosynthesis provoked mini ice ages. In the modern day, we often get rid of our rubbish by storing it underground, from landfill sites to special chemical waste or radioactive materials. And in the United States, there are 144,000 special disposal sites today.
Whatever happens, there will be sacrifices. No geoengineering technique is foolproof: methods are expensive, risky, only so effective, and currently littered with unknowns. Although it should be considered an emergency response, we can’t let the possibility of geoengineering stop us from cutting down emissions and taxing the hell out of those who don’t. What price should we pay to stop climate change?
This article was written by the Things We Don’t Know editorial team, with contributions from AlmeidaJ, Ed Trollope, Jon Cheyne, Cait Percy, Johanna Blee, and Rowena Fletcher-Wood.
This article was first published on 2021-03-05 and was last updated on 2021-03-05.
References
why don’t all references have links?
[1] Climate Geoengineering – Carbon Capture and Storage, Michael Stephenson, p14, Bulletin of the Environmental Chemistry Group, Royal Society of Chemistry, July 2016
[2] Reid, Philip C., et al. Impacts of the oceans on climate change. Advances in marine biology 56 (2009): 1-150
[3] 7. Stephenson, Michael H. Returning carbon to
nature: coal, carbon capture, and storage. Newnes, 2013
[4] Smith, Lydia J., and Margaret S. Torn. Ecological limits to terrestrial biological carbon dioxide removal." Climatic Change 118.1 (2013): 89-103
[5] Salter, Stephen, Graham Sortino, and John Latham. Sea-going hardware for the cloud albedo method of reversing global warming. Philosophical Transactions of the Royal Society of
London A: Mathematical, Physical and Engineering Sciences 366.1882 (2008): 3989-4006
[6] Oleson, K., G. Bonan, and J. Feddema, 2010: Effects of white roofs on urban temperature in a global climate model, Geophys. Res. Lett., 37, L03701, doi:10.1029/2009GL042194
[7] Doughty, C. E., C.B. Field, and A. M. S. McMillan, 2011: Can crop albedo be increased through the modification of leaf trichomes, and could this cool regional climate? Climatic Change, 104, 379–387, doi:10.1007/s10584-010-9936-0
[8] Jones, Andy, Jim Haywood, and Olivier Boucher. Climate impacts of geoengineering marine stratocumulus clouds. Journal of Geophysical Research: Atmospheres
(1984–2012) 114.D10 (2009)
[9] Alan Robock, 20 Reasons Why Geoengineering May Be a Bad Idea, Bulletin of the Atomic Scientists 64 (2008), 14-18
[10] Pitari, Giovanni, Valentina Aquila, Ben Kravitz, Shingo Watanabe, Simone Tilmes, Eva Mancini, Natalia De Luca, and Glauco Di Genova, Stratospheric Ozone Response in Experiments G3 and G4 of the Geoengineering Model Intercomparison Project (GeoMIP), NASA Technical Reports Server (NTRS) ID: 20140011337, 2013
[11] Xia et al., 2014: Solar radiation management impacts on agriculture in China: A case study in the Geoengineering Model Intercomparison Project (GeoMIP). J. Geophys. Res. Atmos., 119, doi:10.1002/2013JD020630
[12] Angel, Roger. Feasibility of cooling the Earth with a cloud of small spacecraft near the inner Lagrange point (L1). Proceeding of the National Academy of Sciences 103.46 (2006): 17184-17189
Recent geoengineering News
Get customised news updates on your homepage by subscribing to articles